GALILEAN RELATIVITY 2

GALILEAN RELATIVITY PART TWO
EVENTS, OBSERVERS, AND FRAMES OF REFERENCE
We begin by defining some important terms. In relativity an event is any occurrence with which a definite time and a definite location are associated; it is an idealization in the sense that any actual event is bound to have a finite extent both in time and in space.
A frame of reference consists of an array of observers, all at rest relative to one another, stationed at regular intervals throughout space. A rectangular coordinate system moves with the observers, so that the x, y, and z coordinates of each observer are constant in time. The observers carry clocks that are synchronized: each clock has the same reading at the same time.
Each observer records all events that occur at her location. Each event has four coordinates: three space coordinates and a time. By definition, the space coordinates are the coordinates of the observer who detected the event and the time of the event is the reading of her clock when it occurs.
A second frame of reference consists of another array of observers, all at rest relative to one another and all moving at the same velocity relative to the first set. They have their own coordinate system and their own (synchronized) clocks, and they also record the coordinates of events. The coordinates of a given event in two frames of reference are, in general, different. The central problem of relativity is just to determine the relation between the two sets of coordinates; this turns out to be not so simple a matter as it first appears.
THE PRINCIPLE OF RELATIVITY AND INERTIAL FRAMES
The principle of relativity was first enunciated by Galileo in 1632. Galileo's argument is clear and graphically put.
Salviatus: Shut yourself up with some friend in the main cabin below decks on some large ship and have with you there some flies, butterflies, and other small flying animals. Have a large bowl of water with some fish in it; hang up a bottle which empties drop by drop into a wide vessel beneath it. With the ship standing still, observe carefully how the little animals fly with equal speed to all sides of the cabin. The fish swim indifferently in all directions; the drops fall into the vessel beneath; and, in throwing something toward your friend, you need throw it no more strongly in one direction than another, the distances being equal; jumping with your feet together, you pass equal spaces in every direction. When you have observed all these things carefully (though there is no doubt that when the ship is standing still everything must happen in this way), have the ship proceed with any speed you like, so long as the motion is uniform and not fluctuating this way and that. You will discover not the least change in all the effects named, nor could you tell from any of them whether the ship was moving or standing still. In jumping, you will pass on the floor the same spaces as before, nor will you make larger jumps toward the stern than toward the prow, despite the fact that during the time that you are in the air the floor under you will be going in a direction opposite to your jump.... Finally the butterflies and flies will continue their flights indifferently toward every side, nor will it ever happen that they are concentrated toward the stern, as if tired out from keeping up with the course of the ship, from which they will have been separated during long intervals by keeping themselves in the air. The cause of all these correspondences of effects is the fact that the ships' motion is common to all the things contained in it.
Galileo is asserting, in effect, that the laws of nature are the same in any two frames of reference that move uniformly with respect to one another. If identical experiments are carried out by two sets of observers, with identical initial conditions, all the results will be the same. It follows that there is no way to determine by means of experiments carried out in a given frame of reference whether the frame is at rest or is moving uniformly. Only the relative velocity between frames can be measured. This set of assertions is called the principle of relativity.
Galileo's motivation was to refute Aristotle's argument that the earth must be standing still. If the earth were moving, Aristotle had claimed, a stone dropped from the top of a tower would not land at its base, since the earth would have moved while the stone was falling. Galileo argues that the earth plays a role entirely analogous to that of the ship in his example; just as a stone dropped from the top of a mast lands at its foot whether the ship is moving or at rest, so does one dropped from a tower on earth. And just as observations carried out within the ship cannot be used to decide whether the ship is standing still or moving uniformly, so the observed motion of objects on earth implies nothing about the motion of the earth other than that it is (approximately) uniform.
Although Galileo may not have carried out all the ship experiments, he definitely performed the falling rock experiment as well as many others on falling bodies. In a famous letter replying to Francesco Ingoli, who had attacked his views and sided with Aristotle, Galileo says, "whereas I have made the experiment, and even before that, natural reason had firmly persuaded me that the effect had to happen in the way that it indeed does." Several remarks are in order concerning Galileo's principle of relativity. First, the observations on which the principle was based were necessarily limited to quite slow speeds. Perhaps if the ship were moving very rapidly, shipborne observers might detect unusual effects that would enable them to conclude that their ship was indeed in motion. If that were to happen, the relativity principle would be only approximately valid. The laws of nature might be (very nearly) the same in two frames of reference that move slowly relative to one another but quite different in two frames whose relative velocity is great. Galileo's observations obviously could not exclude such a possibility, and even today the direct evidence from physics in moving laboratories is limited to fairly low velocities. Indirect evidence, however, strongly supports the hypothesis that the relativity principle holds for any speed.
Galileo's experiments all deal with phenomena in what is nowadays called mechanics; on the basis of those experiments, therefore, one can conclude only that a principle of relativity applies to the laws of mechanics. Perhaps other experiments, involving different phenomena, can distinguish among frames. Nineteenth-century physicists believed that electromagnetic and optical phenomena provide just such a distinction. According to the view prevalent during that period, there exists a unique frame of reference in which the laws of electromagnetism take a particularly simple form. If that were so, the principle of relativity would not apply to electromagnetic phenomena: the results of some experiments would depend on the observer's motion relative to the special frame. Many experiments were performed with the aim of determining the earth's motion relative to the special frame, but they all failed to detect any effect of that assumed motion. The most important was the Michelson-Morley experiment.
For Einstein, it was aesthetically unsatisfying that a principle of relativity should hold for one set of phenomena (mechanics) but not for another (electromagnetism.) He postulated that Galileo's principle applies to all the laws of nature; this generalization forms the basis for special relativity. The relativity principle has an important philosophical implication. If there is no way to distinguish between a state of rest and a state of uniform motion, absolute rest has no meaning. Observers in any frame are free to take their own frame as the standard of rest. Shore-based observers watching Galileo's ship are convinced that they are at rest and the ship is in motion, but observers on the ship are equally entitled to regard them selves as being at rest while the shore along with everything on it moves. The question, Which observers are really at rest? has no meaning if there is no cqnceivable experiment that could answer it. (According to observers in an airplane flying overhead, both shore observers and ship observers are in motion.)
In sum, the principle of relativity denies the possibility of absolute rest (or of absolute motion). Motion can be defined only relative to a specific frame of reference, and among uniformly moving frames strict democracy prevails: any frame is just as good as any other. Any reference to a body "at rest" should be understood to mean at rest in a frame of reference fixed on the earth" (or in some other specified frame).
The distinguishing feature of uniformly moving frames is that in any such frame the law of inertia holds: a body subject to no external forces remains at rest if initially at rest, or if initially in motion, it continues to move with constant speed in the same direction. In an accelerated frame, the law of inertia does not hold. Instead bodies seem to be subjected to peculiar forces for which no agent can be identified. Those forces, called inertial forces, have observable consequences. Observers in a given frame can determine whether their frame is inertial by carrying out experiments to test whether the law of inertia holds. A frame of reference fixed on earth satisfies the criterion fairly closely; for most purposes such a frame can be regarded as inertial. Because of the earth's rotation, however, an earthbound frame is not strictly inertial. Even a frame of reference fixed at the pole, which does not partake of the earth's rotation, is not strictly inertial because the earth is moving in a curved orbit around the sun. And the sun is itself in orbit about the center of the galaxy. An inertial frame is an idealization in the sense that no experiment can assure us that our frame is strictly inertial, that is, that a body subject to no forces does not experience some tiny acceleration.
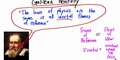
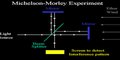
Hope is the bedrock of this nation. The belief that our destiny will not be written for us, but by us, by all those men and women who are not content to settle for the world as it is, who have the courage to remake the world as it should be.
---Barack Obama