There’s a glitch at the edge of the universe that could remake physics
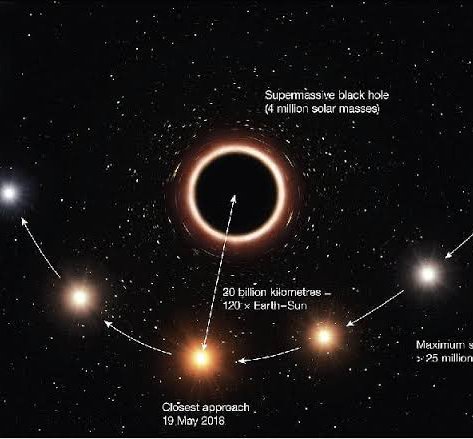
This immutable number determines how stars burn, how chemistry happens and even whether atoms exist at all. Physicist Richard Feynman, who knew a thing or two about it, called it “one of the greatest damn mysteries of physics: a magic number that comes to us with no understanding”.
Now its mystery is deepening. Controversial hints suggest this number might not be the universal constant we had assumed, instead varying subtly over time and space. If confirmed, that would have profound consequences for our understanding of physics, forcing us to reconsider basic assumptions about the structure of reality. While arguments about the true significance of the findings rage, experiments looking both deep into the cosmos and at the fine-grained structure of reality in the lab are now set to deliver a definitive verdict – perhaps.
The idea that constants of nature – things like the speed of light, strength of forces and the masses of various particles – might not be so constant has an illustrious history. In 1937, physicist Paul Dirac wrote to the journal Nature, questioning astronomer Arthur Eddington’s attempts to calculate the constants from scratch. How could we be sure they haven’t changed over cosmological time?
The fine structure constant, also known as alpha, is a case in point. Alpha lies at the centre of a theory Dirac initiated and Feynman worked on: quantum electrodynamics, or QED. This is the quantum theory of the electromagnetic force, and describes the interactions between light and matter. Alpha determines their strength. It is itself constructed from the speed of light, the electron’s charge, pi – few physical theories are complete without pi – and a couple of other fundamental constants, carefully arranged so that it is just a pure number, independent of human influence: 0.00729735, just a whisker away from 1/137.
Change this number by a smidgen, and you change the universe. Increase it too much, and protons repel each other so strongly that small atomic nuclei can’t hold together. Go a bit further and nuclear fusion factories within stars grind to a halt and can no longer produce carbon, the element on which life is based. Make alpha much smaller, and molecular bonds fall apart at lower temperatures, altering many processes essential to life.
A fistful of constants
A large bugbear of physicists is the way our theories of nature require us to inject a set of arbitrary numbers to make them reflect reality. There seems to be no rhyme or reason for these numbers: they are just there, and we must measure them in experiments.
- The standard model of particle physics requires at least 19 such numbers, including the fine structure constant, also known as alpha, the mass of the Higgs boson, and a bevy of others characterising particle masses and interaction strengths.
- To reproduce physics in general, you must add in the gravitational constant (aka “big G”), the speed of light and the Planck constant, which gives the basic size of quantum things.
- The standard cosmological model requires another 12 parameters, including the Hubble constant, which describes the universe’s expansion rate, and factors to do with dark matter and dark energydensities.
On Earth, at least, alpha keeps itself within strict bounds. Lab experiments show that the most it could vary by in our neck of the woods is a few parts per 10 billion. That makes it 100,000 times more accurately pinned down than “big G”, the constant that determines gravity’s strength, for example.
But as Dirac had hinted, perhaps electromagnetic interactions were weaker or stronger in the past, or are different in distant parts of the universe. That could be important at a time when physicists seem to have reached an impasse in their efforts to unveil deeper truths about reality. “We have a basic set of equations that is half a century old and has never been contradicted by any measurement,” says Carlo Rovelli at the University of Aix-Marseille in France. “If we found a measurement that differs from this, it would be a big deal: finally something really new.” Paolo Molaro, who researches variations in constants at the Astronomical Observatory of Trieste, Italy, agrees. “If variations are present, they would reveal new physics,” he says.
That could include the presence of extra dimensions, for example. String theory, one well-backed bet for a next-generation theory of physics, proposes the existence of tiny, curled-up dimensions we can’t see. That has effects on things like alpha. “The status of the quantities we call constants is somewhat downgraded if you believe there are extra dimensions,” says cosmologist John Barrow at the University of Cambridge. “If there are really nine or 10 dimensions of space, with only three large, then the true unchanging constants of nature live in the total number of dimensions and the three-dimensional shadows that we observe are not true constants.”
For physicist John Webb, the possibility of a varying alpha became a matter of near-obsession two decades ago. In 1996, he was a young researcher from Australia visiting Barrow, then at the University of Sussex, UK. The two fell to discussing Dirac’s musings on inconstant constants. Webb wondered whether light collected by some of our most powerful telescopes might settle the question.
Getting a toehold

Some of this light has been travelling for a very, very long time. The Keck telescopes atop Mauna Kea, the highest point of Hawaii, can pick up light emitted by extremely luminous galaxy cores, or quasars, around 12 billion years ago. On its journey to Earth, some of this light has passed through clouds of gas that absorb certain wavelengths. This gives a crucial toehold on alpha. “If you change alpha, you’re changing the degree of attraction between the electron and the nucleus,” says Webb. This changes the wavelengths absorbed by a given atom – meaning the absorption spectrum creates a kind of barcode unique to the value of alpha when the spectrum was created.
With colleagues from the University of New South Wales, Webb developed a new method for analysing these complex absorption spectra, and applied it to Keck quasar data. By 1998, he and his collaborators, including Barrow, had their first results: between 12 and 6 billion years ago, alpha had increased by an average of six parts in a million. It wasn’t enough to significantly affect physics at that time. But it was a change.
This was a sensational result, and very few people believed it – perhaps for good reason. “The search for varying alpha evidence is technically very difficult,” says Barrow. There were only 23 spectra in the analysis and they all came from the Keck telescope, raising the possibility that a systematic error in the apparatus might be skewing the data.
It was the start of a long-running game of cat and mouse. Webb and a changing group of collaborators would publish a fresh analysis showing a variation using new or different data, and some other group would refute the result. Each time, Webb’s team refuted the refutations, while working to find sources of systematic error for themselves. In the meantime, they also gained access to data from another telescope, the Very Large Telescope (VLT) high in the Chilean Andes.
Their latest claim about alpha is that it changes gradually and approximately linearly with distance from Earth. “If we travel a distance corresponding to the distance light has travelled since the big bang, we find ourselves in a part of the universe where physics is just starting to be noticeably different,” says Webb. The universe has been expanding since the big bang, however, so the cosmos extends even further than that. The linear progression suggests that in these invisible regions, alpha could vary enough that the universe itself will start to look very different. “It may be so different there that life as we know it can’t possibly exist,” says Webb.
He admits that this is still highly speculative. The margins of error on the data are big, and the variation might go away with better measurements.
Michael Murphy of Swinburne University in Australia certainly thinks it will. Murphy has worked on alpha for years, and Webb was his PhD supervisor. Other than Webb, no one knows the hardware, data or analysis techniques better. In 2014, Murphy claimed to have finally found an error that quashed claims of variable alpha.
He and Swinburne colleague Jonathan Whitmore realised that light from the lamp used for calibrating their analysis instrument didn’t go through the same twists and turns as cosmic light. When they recalibrated the instrument with light from nearby astronomical bodies such as the sun, sun-like stars or light-reflecting asteroids, it changed the results. “We find it screws up the spectra, shifting some lines towards other lines, and others away from other lines depending on where you are in the spectrum,” says Murphy. That is just enough to create the illusion of a varying alpha.
In 2017, working with Vincent Dumont of the University of California, Berkeley, Webb refuted this. The data analysis is flawed, the pair claim, and applies only to a subset of the results derived from the VLT, not the original results from Keck. Murphy concedes this point, but says his confidence in these results is “undermined”.
He isn’t walking away, however. “We still don’t understand fundamental constants: that motivation has not changed,” he says. “We ought to be trying to measure these things as best we can, and wherever we can. We just have to do things differently – and better – in the future.”
That is already happening. New experiments aim to measure alpha to greater precision in the lab than ever before (see “Change on the ground”). Meanwhile, in November, a new instrument on the VLT will come online, the Echelle Spectrograph for Rocky Exoplanet and Stable Spectroscopic Observation, or ESPRESSO. In Murphy’s estimation it is a dream machine for measuring quasar spectra. “It’s a super-duper ultra-stable spectrograph that will nail this question,” he says.
Not that operating ESPRESSO will be plain sailing, says Francesco Pepe, the instrument’s principal investigator – they will first have to calibrate it and generally get to grips with it. “Our feeling is that by the end of 2019 we will be able to confirm or rule out present claims of variability of the fine structure constant [alpha] at the level of few parts per million,” he says. ESPRESSO should be able to search for variations over the last 10 billion years – maybe more – and probe the whole observable universe. “I think the prospects for significant progress are now really good,” says Barrow.
Webb, meanwhile, is going blind, scientifically speaking. Coming up with a value of alpha from quasar spectra involves subjective decision-making about which parts of the spectra to include. Sometimes it is tempting to ignore regions of the spectrum that aren’t “interesting” because they have too few absorption lines, but these simplifications could skew the results. To avoid this, Webb and student Matthew Bainbridge have created a machine-learning algorithm that takes a thorough, objective look at the raw data. “We’ve changed all the manual decision-making and thrown the problem at an array of supercomputers,” says Webb.
He will only look at the results once the machine has crunched 1000 measurements, a “sealed envelope” that removes the temptation to downplay individual measurements that don’t fit the hypothesis. There are around 500 measurements currently in the bag, and the work should be completed around the same time as the ESPRESSO analysis, says Webb.
Then, whatever the outcome, he will walk away. “Time goes on, and there are other things you want to do in life,” says Webb. “I’ll work on this until the end of 2020, see what the results are, and let somebody else take over.” He doesn’t think the question of the inconstant constants will be settled, even then. “It started with Dirac in 1937; it’s not going to suddenly end in 2020,” he says. “But it can become somebody else’s concern.”
Change on the ground
Controversial as it is, John Webb’s pioneering work on variations in the constant alpha has brought into the mainstream the idea that the universal laws of nature might not be constant, but vary in space and time.
In June this year, Charles Clark at the US National Institute of Standards and Technology and his colleagues laid out a scheme of lab experiments that could look for changes in physics constants. One is simply to synchronise two atomic clocks and watch to see if that synchronisation drifts over time.
The experiments could provide a gold standard against which any future claims of variations could be compared, and provide clues to help physicists go beyond the standard model of particle physics, our current most coherent description of how the universe works. “We know that the standard model has to fail somewhere,” says Clark. “Variations would be inconsistent with prevailing theories of physics.”
Earlier this year the experiments provided the most accurate measurement of alpha on Earth yet. Holger Müller and his colleagues at the University of California, Berkeley, watched interactions between photons and caesium atoms to pin alpha’s value down to better than one part in a billion. That is close to ruling out certain suggestions for post-standard-model physics. And there is more to come. “We are building a new experiment and believe we can gain an order of magnitude in the next few years,” says Müller.
But Webb thinks terrestrial experiments are barking up the wrong tree. Any changes in alpha are probably only detectable over cosmological time scales, he says. “It could be, for example, that changes were rapid in the early universe, but today there is little or no change,” says Webb. “If that’s the case, ground-based experiments will never detect a change, no matter how precise.”
This article appeared in print under the headline “The wrong number”

I have no special talent. I am only passionately curious.
----Albert Einstein